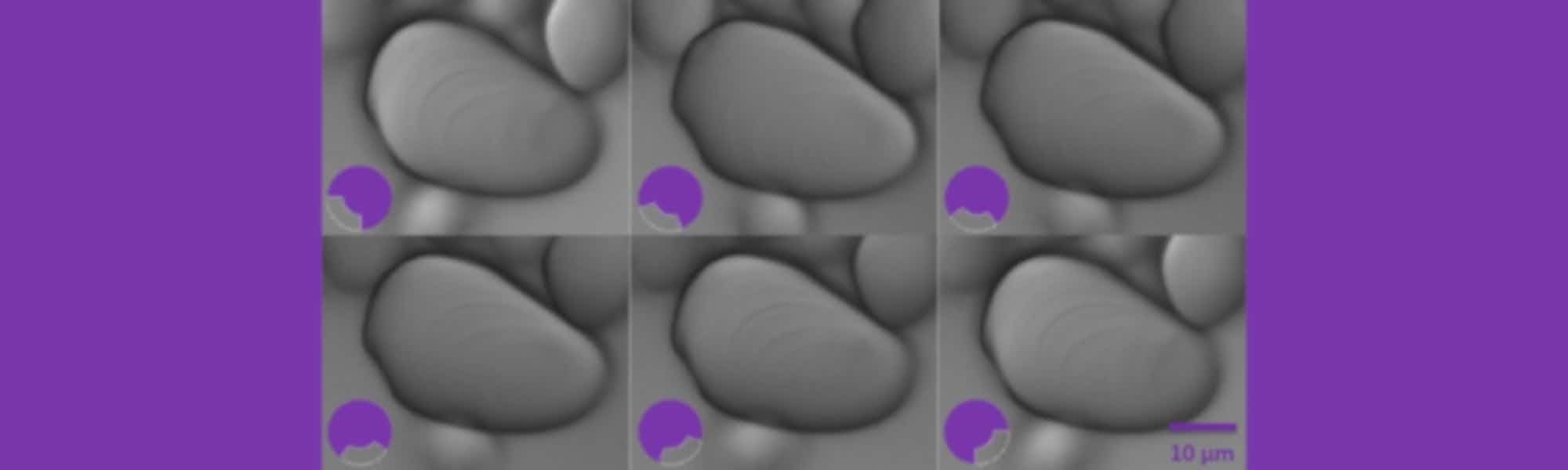
A guide to Dodt Gradient Contrast
Dodt Gradient Contrast (DGC) is a microscopy technique developed by Professor Hans-Ulrich Dodt during the 1990s while working at the Max Planck Institute of Psychiatry in Munich.
By using a similar principle to oblique contrast, DGC produces images with contrast comparable to those made by Differential Interference Contrast (DIC) and without the imaging artefacts associated with other techniques like phase contrast.
Like IR-DIC (infrared DIC), DGC is a form of infrared videomicroscopy (although visible wavelengths can also be used) that improves the contrast of images of unstained tissue (e.g. brain slices) in comparison to brightfield microscopy.
How it works
In DGC, a gradient contrast tube is placed between the light source and the condenser. Within the tube, there is a lens that focuses the light towards a diffuser positioned a short distance after a quarter annulus. The annulus blocks some of the light, and the diffuser creates a gradient of illumination. A second lens then collimates this light before it enters the condenser (see figure 1). The difference in intensity across edges in the sample enhances interference in the light path turning the phase shift through the sample into a change in amplitude.
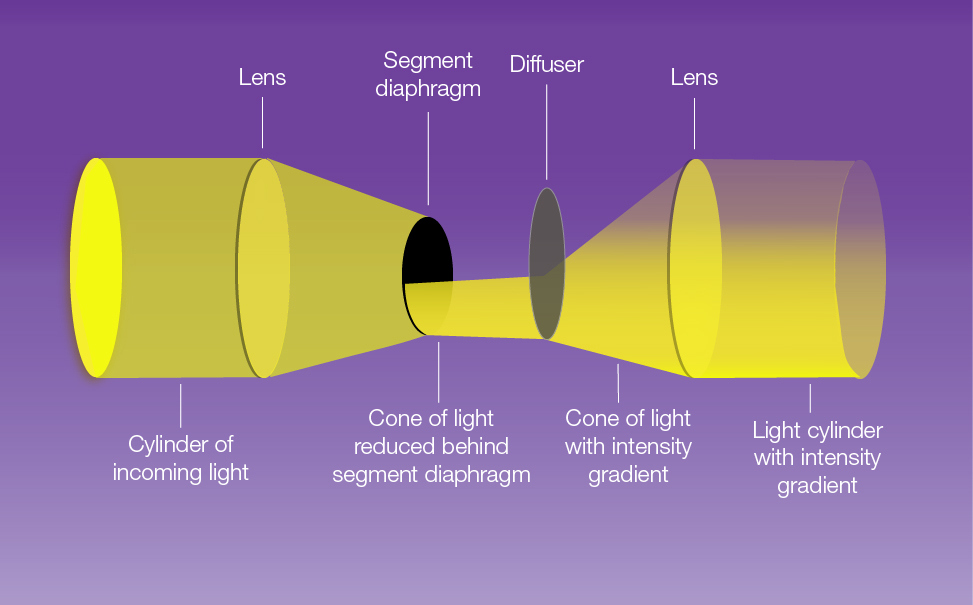
This method allows some light to illuminate the entire area initially lit by the light cylinder, making the image comparable to the actual object and reducing optical artefacts.
Because much of the light is blocked, DGC generates less stray light at the sample, enabling the use of a 1.4 numerical aperture (N.A.) oil condenser. Additionally, the curved slit of the quarter annulus means that the gradient of illumination occurs in two perpendicular directions. This feature becomes advantageous when attempting to visualise the dendritic branches of neurons travelling in various directions.
Setting up gradient contrast
The only additional components required to make a brightfield microscope into a scope capable of DGC are the gradient contrast tube itself and potentially a high-intensity infrared light source. The tube is placed between the transmitted light source and the transmitted illumination port of the microscope.
After installing the contrast tube, the first thing to do is to set up Koehler illumination on the microscope. Additionally, if your microscope has an additional diffuser between the illumination port and the condenser this must be removed, as it will dilute and disperse the contrast output provided by the spatial filter.
Once the Gradient Contrast Tube is installed and the microscope is properly set up for Koehler illumination, the microscope can be optimised for Dodt Contrast (See figure 3 for schematic diagram of an upright microscope set up for DGC). The spatial filter adjustment ring (A) can be rotated to adjust the position of the quarter annulus by approximately 90 degrees enabling you to change the direction of the gradient across the sample. Finding the best placement for ring A will depend on your sample and is best optimised by trial and error. The direction of the gradient will highlight structures running anti-parallel to the spatial filter.
The diffuser position adjuster (B) can be rotated to move the diffuser axially along the light path (closer or further from the microscope) to alter the amount of contrast on the image by increasing or decreasing the sharpness of the gradient.
As an example, Figure 4 shows starch granules obtained from potato starch (Solanum tuberosum) observed without a Dodt contrast tube. The starch granules appear to be smooth objects without much definition beyond the outline.
However, with the insertion of the gradient contrast tube, the growth rings (or lamellae) can be observed thanks to the increased contrast (Figure 5).
By rotating the quarter annulus using ring A, it is possible to see the outline of the growth rings in their different positions as they are radially oriented. These layers accumulate around the hilum, which is the granule’s point of botanical origin.
Adjusting the position of the diffuser with ring B alters the amount of contrast on the image (Figure 6). In the right panel of Figure 6, the position of the diffuser closer to the light source enables the observation of the hilum as a slightly depressed zone (purple arrow).
If after adjusting the spatial filter and diffuser you are still unable to obtain a clear image, a further method that can be employed to enhance the contrast is to laterally offset the condenser and bring the shadowed edge of the iris into the field of view to create additional oblique illumination within the light path (do not move the condenser axially). This will cast a gradient of light across your field of view (from darker to lighter), which you can adjust by altering the light source intensity. This process should enable you to obtain an enhanced contrast image in the centre of your field of view.
Please note that the Scientifica gradient contrast tube can be installed without any particular radial orientation. Therefore the absolute position of the spatial filter will change depending on the alignment of the tube when afixed to the microscope. Additionally, the optimum position of Rings A and B will depend on the experimental sample.
Advantages
As you can see from figures 5 and 6, DGC leads to images similar to DIC. The technique makes unstained living tissue visible in a useful way without harming it.
DGC is a cost-effective contrast method that does not require the purchase of expensive optical elements. Additionally, no further elements are added into the light path between the sample and camera or detector, making it an ideal contrast technique for patching in combination with two-photon excitation fluorescence microscopy.
With a good camera, the fast frame rates available with this approach also makes it an excellent tool for positioning micropipettes during patch clamp experiments to enable patch-clamp electrophysiology in thick brain slices (<300 µm).
Limitations
The amount of light lost through the quarter annulus is significant. This loss can lead to dark images which will, in turn, cause a lack of contrast. This loss can be exacerbated when using an LED which has a lower maximum power output than a traditional arch discharge illumination source.
DGC is not as simple as other contrast techniques to align and set up correctly, the requirement to readjust the settings regularly can be an annoyance to some users.
Applications
The primary use of DGC is to visualise unstained neurons, which are mainly phase objects i.e. they alter the phase of the light waves that pass through them but not their amplitude. DGC is particularly useful when attempting to observe neurons in thick brain slices.
DGC is also ideal for performing patch-clamp experiments when used in combination with two-photon illumination as there is no requirement to have any optics between the collection module placed after the objective lens.
Scientifica have created a printable PDF guide for microscope users. Download it here: