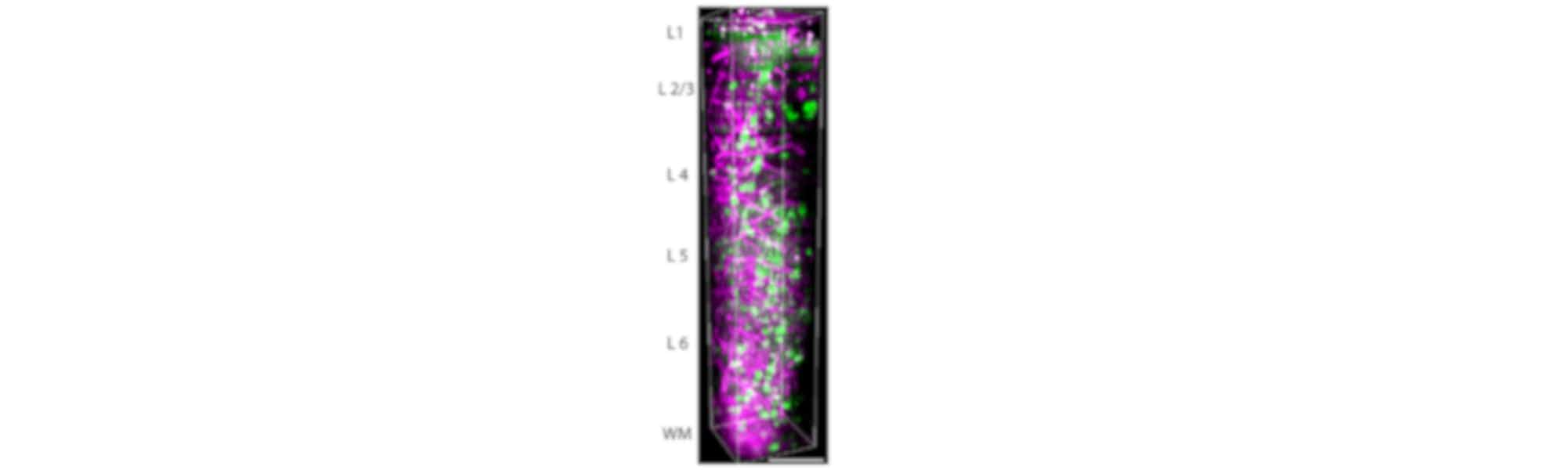
Development of a custom-made three-photon microscope to perform functional imaging in awake animal models
Murat Yildirim, Picower Institute for Learning and Memory
Deep tissue imaging has been my great passion since my doctoral studies. During my PhD, I developed two- and three-photon systems to perform deep tissue imaging in ex vivo tissue samples as well as precise tissue ablation. Then, I realised the possible applications of two- and three-photon microscopy in brain imaging, thus I began a joint collaborative project with Mriganka Sur and Peter So at MIT. Since then, I have been learning to ask fundamental questions in neuroscience and developing multiphoton systems to find the answers to these questions.
How was Scientifica's SliceScope used to build the three-photon system?
When I started to design my scope, one of the first things that I was searching for was a translational stage for moving the sample in lateral and axial directions. Since we knew we did not want to move the animal, especially in the axial direction, I needed two separate stages for lateral and axial movements. Another important criterion in stage selection was not having any electrical noise appearing in images acquired by the photomultipier tubes (PMTs).
For the noise issue, I consulted a couple of my colleagues who have performed various electrophysiology experiments with slices in our lab and they recommended I use Scientifica stages. I purchased Scientifica’s translational stages and SliceScope, and designed the rest of my system around these stages. Since the Slicescope has enough space in the axial direction, I could design my system both for small and big animal experiments.
What has been investigated using the system?
Developing a custom-made three-photon microscope requires optimising laser parameters such as repetition rate and pulse width as well as microscope parameters such as aberration, excitation and emission path optics. By optimising these parameters, we believe that we can make novel discoveries that were not possible before.
I have been utilising this custom-made three-photon system for investigating a variety of biological phenomena in different biological systems. In the recent study that we published in Nature Communications, we looked at evoked neuronal responses of all cortical layers as well as the subplate of the primary visual cortex (V1) in awake mice, while mice were presented with sinusoidal visual gratings. Our measurements of physiological responses and tissue-damage thresholds define pulse parameters and safety limits for damage-free three-photon imaging. To reveal the safety limits for laser pulse energy to avoid any saturation of the Gcamp6s fluorophore, optical breakdown or any physiological changes which may occur without any visible damage, we varied pulse energies on the focal plane from small to high levels, and measured evoked neuronal responses across all layers of the visual cortex and in the white matter. We found that it was safe to image with energy pulses in the range of 0.5–5 nJ in our experimental conditions in each layer for avoiding visible damage or saturation of the chromophore. When the laser energy was between 5 and 10 nJ, saturation of GCaMP6s was initiated and the entire field of view was gradually saturated. Finally, optical breakdown was observed when the pulse energy was >10 nJ. Furthermore, we studied the evoked neuronal responses of each layer while the pulse energy was <5 nJ. We classified laser pulse energies into two subcategories: (i) pulse energies between 0.5 and 2 nJ, (ii) pulse energies between 2 and 5 nJ. We compared both preferred orientation and average calcium signal intensity (ΔF/F) in these two pulse energy groups. Across all layers, approximately 70% of the visually responsive cells preserved their preferred orientation in both of these energy groups. However, the mean response intensity at the preferred orientation was significantly reduced in the second pulse energy group compared to the first in cells with similar preferred orientation. This result was consistent across all layers. Thus, it is crucial not to exceed the 2 nJ pulse energy threshold for evoked neuronal response studies with three-photon microscopy, with similar laser parameters as used by us.
After determining the parameters for safe physiological recordings, we performed functional recordings in all layers of the visual cortex. We found that deep layer neurons such as layer 5 and layer 6 had distinct visual responses to the same visual stimulus. For example, we found that layer 5 neurons are broadly tuned for orientation compared to neurons in other cortical layers. Layer 5 neurons have the highest spontaneous activity among neurons from all cortical layers, this may contribute to their broader tuning property. Another difference between neurons in layer 5 and other cortical layers is the high density of subcortical projection neurons in this layer. In addition, layer 6 neurons have slightly sharper orientation tuning than neurons in other layers. This is consistent with layer 6 containing substantial numbers of corticothalamic projection neurons which have been described as highly orientation selective.
As well as recording from all layers of the visual cortex, we also imaged neuronal responses in the subplate of awake mice V1 for the first time. These neurons are known to have a crucial role in the formation of thalamocortical and intracortical connections. While most subplate neurons seem to be transient and disappear after the cortex has formed, a small population remains, and has been termed as persistent subplate cells. So far, subplate neurons in the mature brain have not been studied at all due to technical challenges of imaging these cells in vivo. A majority of subplate cells in our study are poorly responsive visually and the visually driven neurons have lower visual responses compared to neurons in other cortical layers.
What will be investigated in the future?
So far, my studies have involved imaging evoked neuronal responses of unspecific neurons in awake mice with simple visual stimuli. I have started to perform more cell-specific recordings in different contexts where animals are more motivated to perform goal-directed tasks. Eventually, I would like to record and manipulate these deep layer neurons depending on their input and output as well as understand their contribution to animal performance in specific tasks.
Scientifica SliceScope
A stable and compact upright microscope. The modular design makes the SliceScope a perfect foundation for many different configurations including in vivo or in vitro electrophysiology, multiphoton imaging and confocal imaging.
Banner image: 3D representation of cortical layers of the mouse primary visual cortex (V1), courtesy of Murat Yildirim
Paper reference:
Yildirim, M., Sugihara, H., So, P T C., and Sur, M. Functional imaging of visual cortical layers and subplate in awake mice with optimized three-photon microscopy. Nature Communications (2019) doi: https://doi.org/10.1038/s41467-018-08179-6
Find out about Scientifica's latest product releases, company news, and developments through a range of news articles, customer interviews and product demonstration videos: