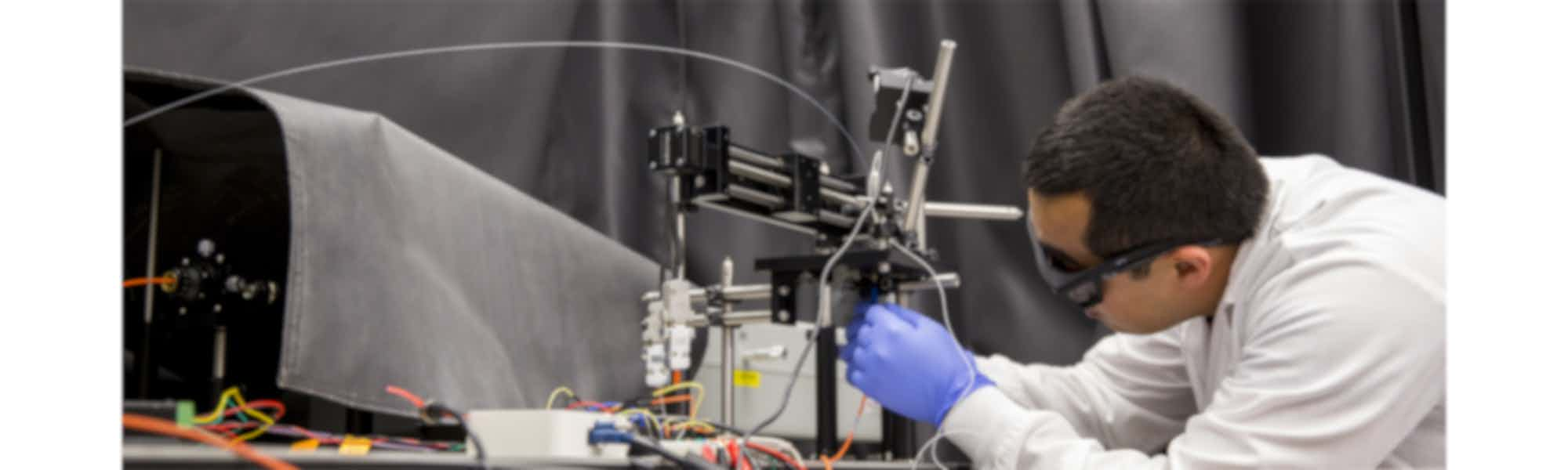
Using Scientifica’s PatchStar for photoacoustic guided electrophysiology: A novel technique for accurate and automated cellular navigation in the deep brain
Patch clamp electrophysiology uses glass micropipette electrodes to stimulate and record from individual neurons, both in vitro and in vivo. Currently, there is no way to target specific cells of interest deeper than 800microns past the cortical surface with any type of imaging tool. The lab of Professor Barbara Smith is the first to incorporate a real-time, photoacoustic feedback system into traditional micropipette electrodes to target, stimulate, and record neurons deep within the living mammalian brain using the PatchStar micromanipulator.
How the photoacoustic effect works
When a molecule absorbs light of a specific wavelength, known as optical absorption, it becomes excited and has a slight thermal expansion. This causes the molecule to increase in size and emit a small ultrasound wave. It is this wave of sound that can be detected. Therefore, specific molecules of interest can be selectively targeted using the photoacoustic effect, by using specific optical wavelengths.
How the researchers used the photoacoustic effect
The Smith lab researchers use a standard glass micropipette as an optical and acoustic waveguide. Light that enters the micropipette travels down the entire length, and is concentrated on to a specific location after exiting the tip of the micropipette.
When the light is absorbed by the target molecules, the molecules become excited and generate a photoacoustic signal. The resulting ultrasound produced by the photoacoustic effect, travels back along the micropipette and is detected by a transducer at the back end of the pipette. A micropipette that is able to release light and detect the generated photoacoustic signal is known as a photoacoustic micropipette (PMP).
The photoacoustic signals that are detected through the PMP disclose information about the presence and location of cellular and molecular targets. This photoacoustic feedback can then be used for autonomous robotic movement of the PMP towards the target.
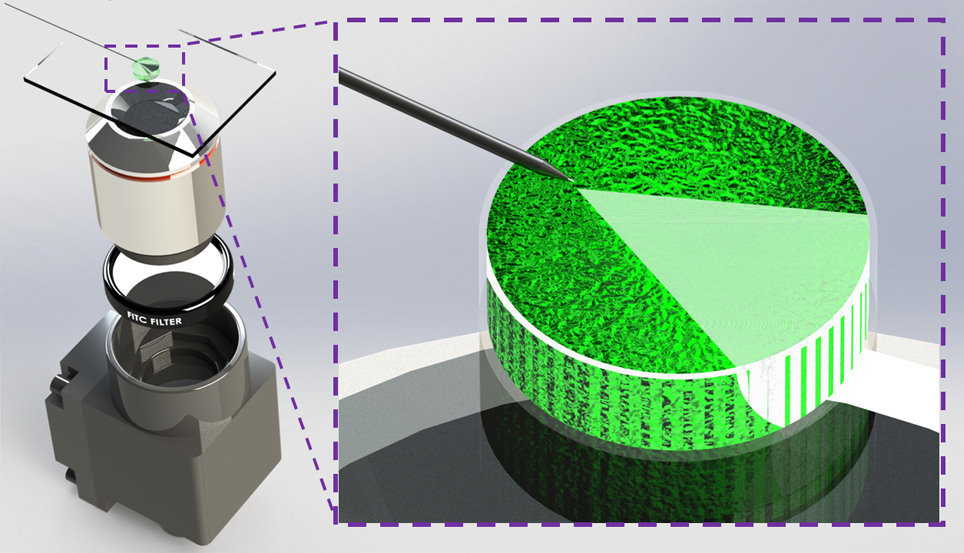
As the photoacoustic target approaches or recedes from the PMP tip, a corresponding increased or decreased photoacoustic signal is generated. Initial studies performed by lead PhD student, Christopher Miranda, showed semi-automated targeting and approach of the PMP through photoacoustic feedback signal intensity, while moving the stage in decreasing vertical steps of 50mm, 10mm and 1mm step sizes. A noticeable increase in photoacoustic signal was detected as the PMP neared the photoacoustic target. This showed the researchers that the PMP can be robotically guided to targets of interest through photoacoustic feedback intensity. This is useful for both in vitro and in vivo applications.
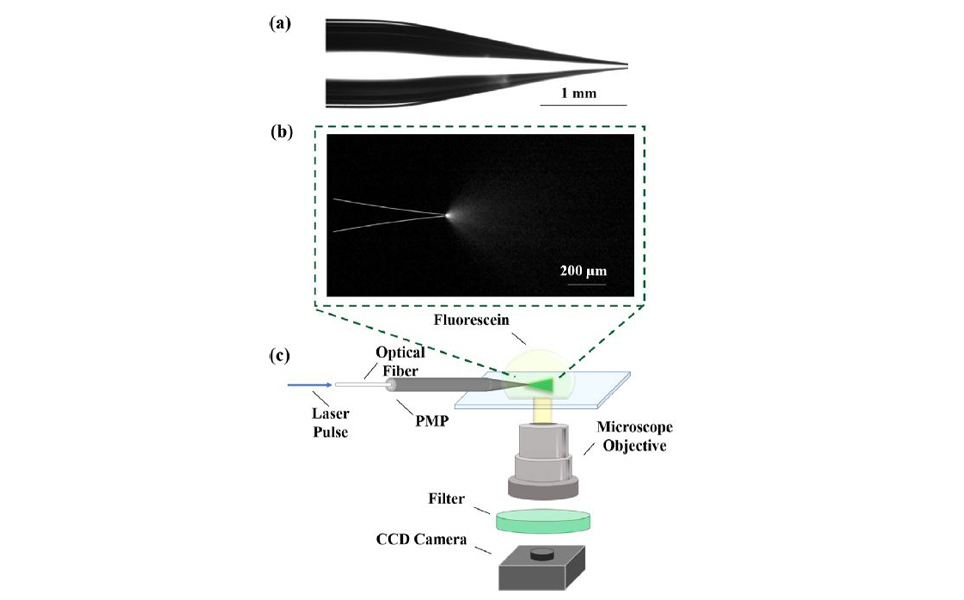
What can photoacoustic guided electrophysiology be used for?
- Photoacoustic guided electrophysiology, first developed in the Smith Lab, seeks to break down barriers for the accurate and automated targeting of neurons at depths beyond what is currently possible.
- Combining the PMP technology with the PatchStar micromanipulator enables autonomous robotic navigation for controlled, real-time cellular identification, stimulation and recording; to uncover fundamentally new biology related to the behavior of single cells and neural networks in the deep brain.
- This technique will significantly improve cell specific navigation to deep brain structures while reducing the technical and expertise barriers to implementation.
- This technique will enable specific cells of interest to be patched and unwanted cells to be avoided.
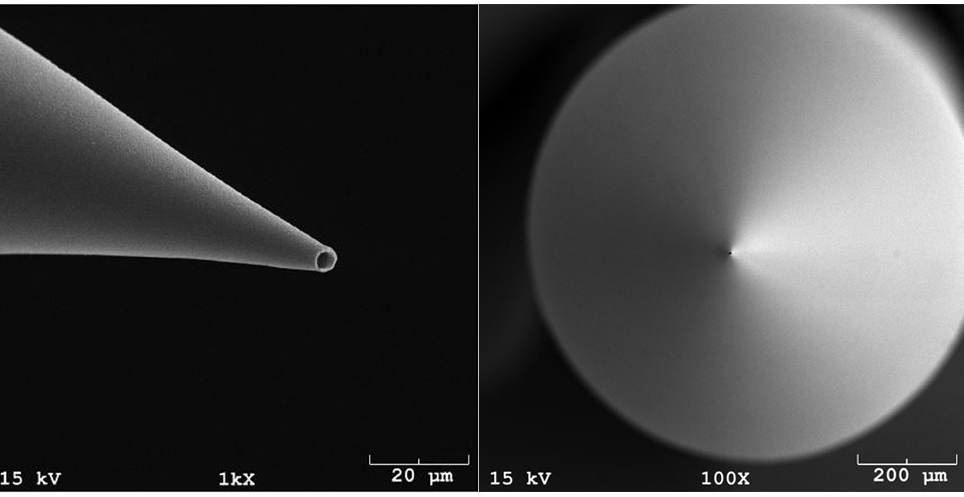
Why was the PatchStar micromanipulator chosen?
The PatchStar micromanipulator was chosen for this research due to its simple and straight forward programming interface through control commands, enabling easy PMP robotic automation. Additionally, the small (100nm) step sizes were extremely beneficial in the development of this technique. Overall, the high resolution of the PatchStar will allow for precise control of the PMP during in vivo studies.
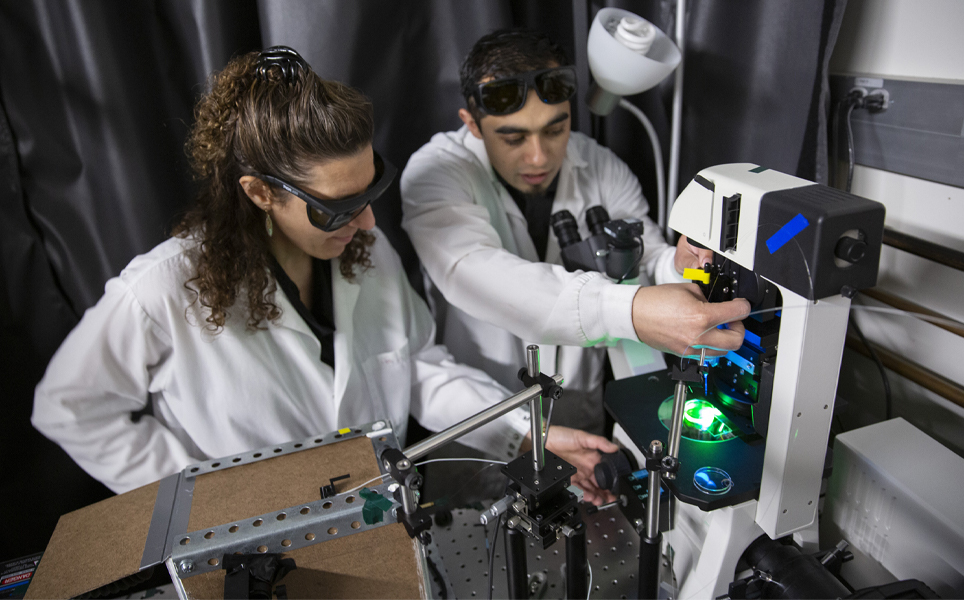
Scientifica PatchStar Micromanipulator
The PatchStar micromanipulator is the most versatile micromanipulator for electrophysiological studies. It is ultra-stable, with less than 1 µm drift over 2 hours for long-term experiments, easily programmable and 20nm resolution for absolute positioning.
What is being investigated next?
Researchers in the Smith lab are now applying this unique PMP technology for select applications in the deep brain.
Sign up to receive our latest news
Find out about Scientifica's latest product releases, company news, and developments through a range of news articles, customer interviews and product demonstration videos.