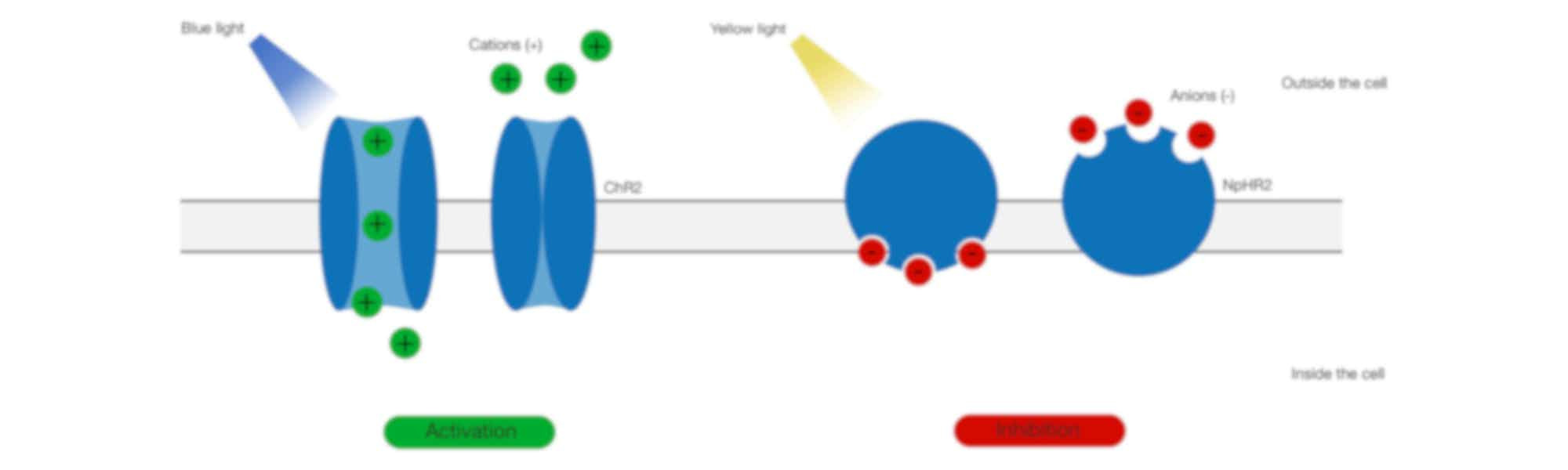
Advances in neuroscience techniques: Optogenetics, chemogenetics, CLARITY and CRISPR
By Deepanjali Dwivedi, PhD
The field of neuroscience has witnessed substantial scientific advances over the last couple of decades, making it feasible for neuroscientists to answer a wide spectrum of questions that were not previously possible. The field has come a long way, from recording action potentials in the giant squid axon to specific connectivity mapping and manipulations. Modern techniques provide better insight into brain functioning, further extending our therapeutic approaches to different neurological anomalies. Here we discuss some techniques that have revolutionised neuroscience.
Optogenetics
Optogenetics involves the expression of genetically encoded photoactivable bacterial opsin proteins on neuronal surfaces to induce neuronal activity. Oesterhelt and Stoeckenius, in 1971, discovered bacterial rhodopsin, which upon optical stimulation can undergo a conformational transition and conduct an ionic current. Channelrhodopsin (ChR) was first used tp change the membrane potential of mammalian cells in 2003. Further developments saw the first-time use of opsins to stimulate neuronal activity in 2005. ChR can pump positive ions into the cell and cause depolarisation, while halorhodopsin (NpHR) can pump chloride ions into the cell and cause hyperpolarisation.
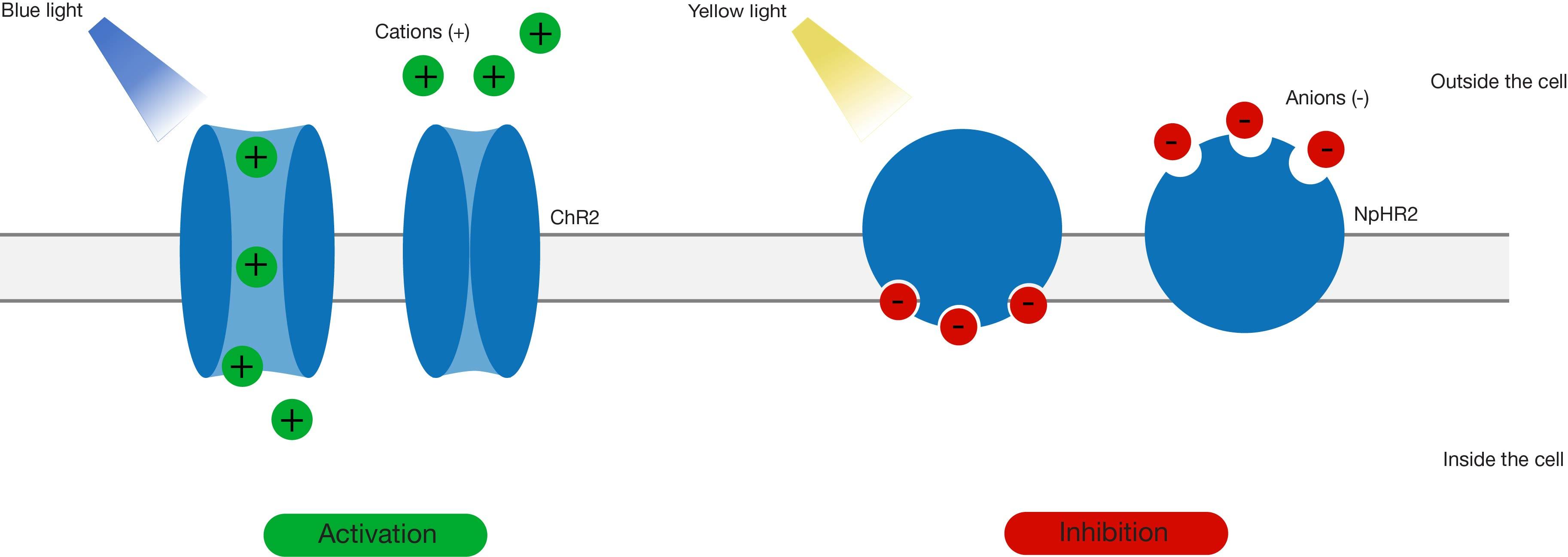
Find out more about optogenetics here
Advances in genetic engineering have contributed to the discovery and generation of multiple variants of these primary opsins which have faster kinetics, a greater range of activation wavelengths and different conducting ions, further expanding the scope of the technique. These rhodopsins can be restricted to specific regions/cells by:
- The adoption of a cre-lox system (where cre recombinase is expressed and active in distinct brain regions or cell types, activating the expression of genes under lox control that encode rhodopsin)
- Viral vectors injected in specific regions
- Genetic linking of the rhodopsin genes to distinct cell type specific promoters, producing a significant level of spatiotemporal specificity.
This technique can be utilised to investigate neuronal connections between different brain regions, the role of various brain regions in specific behavioural paradigms, and various neurological disorders. The technique has become particularly popular and is preferable over electrical stimulations or pharmacological manipulations, because of its high spatial and temporal specificity and a greater extent of possible manipulations. However, a caveat of the technique is the invasive implantation of optical fibres to stimulate in-vivo neuronal activity, which can be damaging to the brain if they are not thin enough.
Chemogenetics
Chemogenetics involves the expression of Designer Receptors Exclusively Activated by Designer Drugs (DREADDS) on the neuronal membrane. Injection of otherwise inert ligands into the animals can activate these designer receptors, leading to enhanced/reduced neuronal activity. Like optogenetics, this technique can yield a high spatial specificity through the use of cell-specific promoters, but unlike optogenetics, it cannot drive neuronal activity as millisecond precision is required. DREADDS are modified GPCRs, such as muscarinic receptors, which bind to clozapine-N-oxide (CNO), a compound that has no biological activity in naïve tissues but activates these receptors. Different DREADDS can be applied to both stimulate and suppress activity of a neuron. Protein engineering has led to bistable DREADDS, which can activate or inhibit a neuron when exposed to the designer drug, and then remain active even in the absence of that drug.
Cre dependent viral vectors or transgenic animals that express DREADDS under promoters can be used to target specific neurons. Researchers can deliver ligands for DREADDS into the animal using a systemic route (intraperitoneal or intravenous injection), by microinjection, addition to feed or water, or infusion via implanted cannulae. This technique can be preferable over optogenetics as it is less invasive and can produce increased neuronal activity for prolonged durations. However, the major caveat of the technique is its non-selective activation due to the limited viral specificity and altered effects on neuronal activity caused by the metabolic products of the ligand.
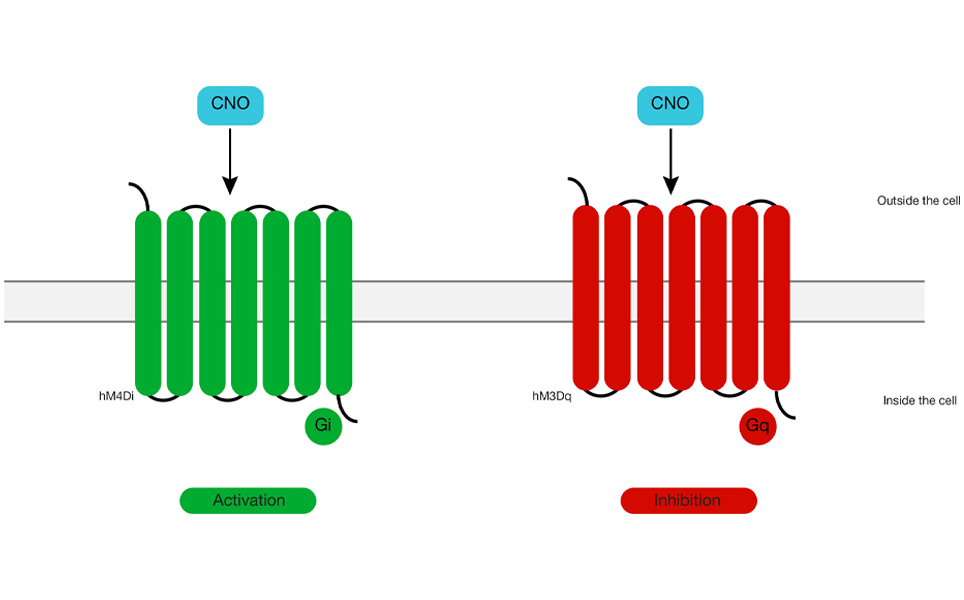
CLARITY
Clear Lipid exchanged Acrylamide hybridised Rigid Imaging Tissue Hydrogel (CLARITY) is a tissue clearing technique that replaces the existing lipid with acrylamide, creating a tissue-hydrogel transparent sample, while preserving the molecular and anatomical organisation of proteins and nucleic acids. Chung and Deisseroth developed this technique in 2013 and it has revolutionised the neuroimaging field. The notable advantage of the technique is that the tissue cleared by CLARITY can be utilised to probe for multiple molecules in the same sample without damaging the tissue and can be stored for later use.
By employing this technique, we can investigate long-range neuronal connections between different regions in intact tissue, which was not attainable before. The primary disadvantage is that it is an expensive, tedious process that causes loss of proteins during sample preparation and repeated imaging.
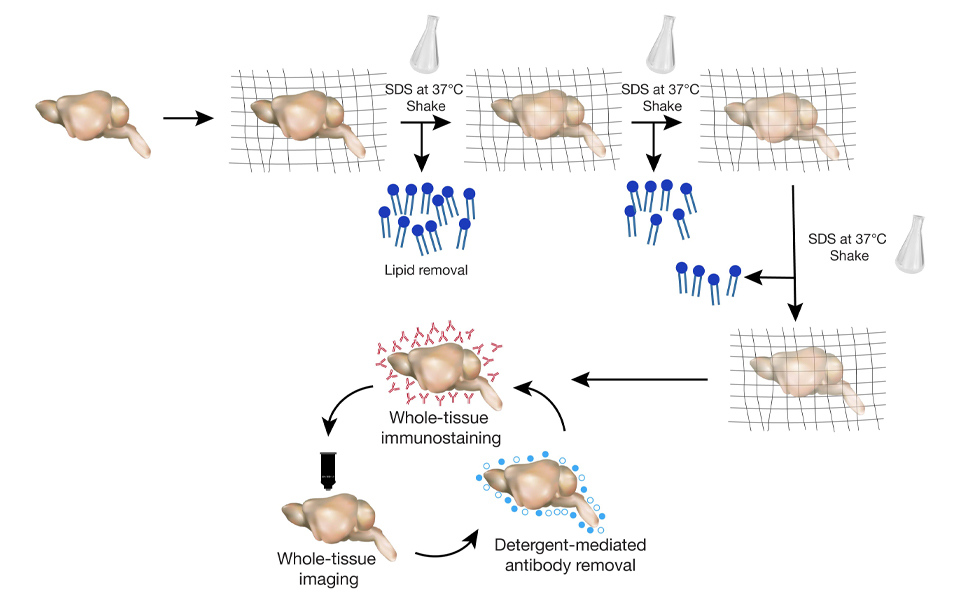
CRISPR
Clustered Regularly Interspaced Short Palindromic Repeats (CRISPR) and CRISPR-Associated Protein 9 (Cas9) is a genome-editing technique that gained fame because of its high precision and efficiency with reasonable economic costs. CRISPR- Cas9 action was first observed in 1987 by Ishino et al. in bacteria and archaea as part of their defence mechanism.
Advances in the same technique can now mediate targeting (by CRISPR), cleaving of the DNA fragments (by Cas9) and repair by the host’s DNA machinery by non-homologous end joining (NHEJ) which generates non-specific mutations or by homology-directed repair (HDR) which introduces specific mutations using the donor template. However, the prime fault of the technique is its off-target action, contributing to unwanted mutations. Choosing a stronger target site with high GC content (these base pairs have stronger bonds which only a high specificity guide RNA can displace), use of lower quantities of Cas9 enzyme, use of guide mRNA/ protein instead of a plasmid with guide RNA which permits a longer time for expression and use of paired guide RNA’s, are some approaches to scale down the off-target effect issue of CRISPR-Cas9 technology.
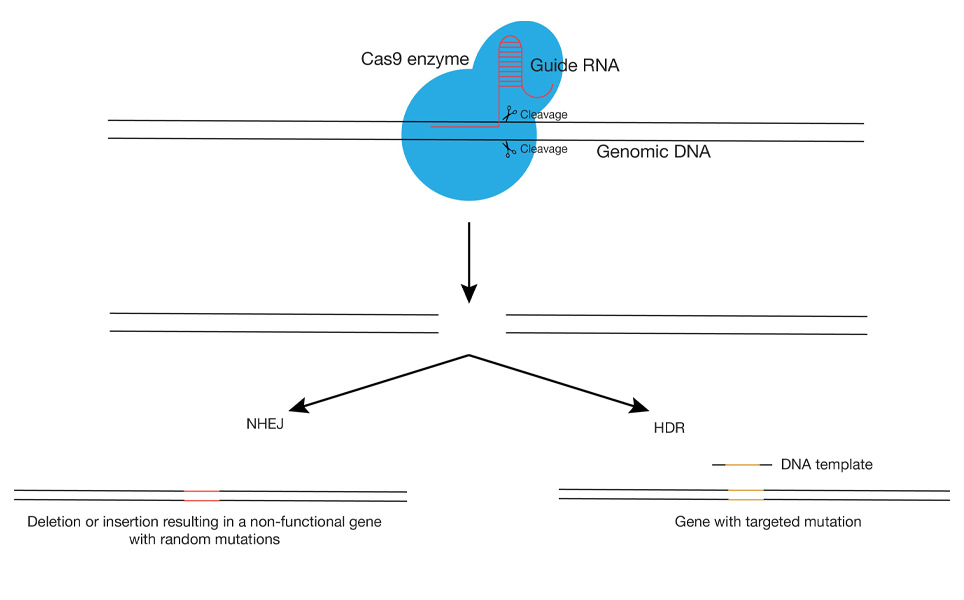
The procedure has found comprehensive implementation in research and has been used for gene editing in several models including cell lines, zebrafish, C. Elegans, drosophila, rodents, and primates. It is now being applied to medicinal treatments, where editing somatic cells of patients may be able to treat various disorders. This gene editing can be used to create mutations (to study specific neurological diseases), to add functional genes in loss of function genetic disorders (to cure diseases), and even to edit plant genomes for the generation of improved quality food products.
Optogenetics, chemogenetics, CLARITY and CRISPR have led to exciting discoveries and therapeutic developments in neuroscience. Either discovered in nature, or developed by scientists, these are now used by researchers around the world to genetically manipulate, target and investigate neurons, neuronal connections and neurological disorders.
Deisseroth K. Optogenetics: 10 years of microbial opsins in neuroscience. Nat Neurosci. 2015;18(9):1213-1225. doi:10.1038/nn.4091
Boyden ES, Zhang F, Bamberg E, Nagel G, Deisseroth K. Millisecond-timescale, genetically targeted optical control of neural activity. Nat Neurosci. 2005;8(9):1263-1268. doi:10.1038/nn1525
Campbell EJ, Marchant NJ. The use of chemogenetics in behavioural neuroscience: receptor variants, targeting approaches and caveats. Br J Pharmacol. 2018;175(7):994-1003. doi:10.1111/bph.14146
Du H, Hou P, Zhang W, Li Q. Advances in CLARITY-based tissue clearing and imaging. Exp Ther Med. 2018;16(3):1567-1576. doi:10.3892/etm.2018.6374
Chung K, Deisseroth K. CLARITY for mapping the nervous system [published correction appears in Nat Methods. 2013 Oct;10(10):1035]. Nat Methods. 2013;10(6):508-513. doi:10.1038/nmeth.2481
Ma Y, Zhang L, Huang X. Genome modification by CRISPR/Cas9. FEBS J. 2014;281(23):5186-5193. doi:10.1111/febs.13110
About the author
Deepanjali Dwivedi is a recent PhD graduate from the National Centre for Biological Sciences (NCBS), India. She has extensive experience in whole-cell patch-clamp electrophysiology and data analysis using MATLAB. She is interested in ion channel physiology, neurological disorders, Microglia, Astrocyte biology, and Interneuron functioning.
Sign up to receive our latest news
Find out about Scientifica's latest product releases, company news, and developments through a range of news articles, customer interviews and product demonstration videos.